If you’ve ever flown on a commercial aircraft, you’ve experienced a remarkable feat of engineering without even realizing it: the complex system of pressurization that keeps you comfortable—and, more importantly, alive—at cruising altitudes where the air is thin and the temperatures are frigid. But why is pressurization necessary, and how does it work? Let’s take a closer look at the science and technology that make high-altitude flight possible.
The Atmosphere: It’s Not Just About the Cold
We all know that temperatures drop as we ascend to higher altitudes. But temperature isn’t the only thing that changes—the air pressure also decreases. At sea level, the atmosphere exerts a pressure of approximately 1013 millibars (approximately 14.7 pounds per square inch). As you climb, this pressure drops dramatically. By the time you reach the cruising altitude of a typical airliner, around 35,000 feet, the pressure is less than a quarter of what it is at sea level.
How Air Pressure Affects Oxygen Absorption
Under normal conditions, oxygen transfers from the air in our lungs to our blood, where it’s carried to the rest of the body. This process depends on adequate air pressure. At lower pressures—like those found at high altitudes—oxygen transfer becomes less efficient, making it harder for your body to get the oxygen it needs. This is why even walking up a hill can feel like a strenuous workout when you’re in the mountains.
Why Aircraft Need to Be Pressurized
As altitude increases, the availability of oxygen decreases. Without pressurization, passengers and crew would quickly experience hypoxia—an oxygen deficiency that can lead to confusion, unconsciousness, and even death. Pressurization allows aircraft to maintain an internal environment similar to the atmospheric pressure at around 6,000-8,000 feet, even when the plane is flying at much higher altitudes.
Aircraft as Pressure Vessels: Why You Can’t Open the Doors Mid-Flight
Aircraft cabins are essentially pressurized vessels, with the internal pressure kept significantly higher than the outside pressure. This is why you can’t open the doors mid-flight—they’re designed to open inward first. With the difference in pressure, the force required to push the door open is far beyond human capability. So, rest assured, you won’t be sucked out of the plane if someone tries to open the door!
Cabin Altitude vs. True Altitude
Here’s where things get interesting. Although an aircraft may be flying at an altitude of 35,000 feet, the pressurization system keeps the cabin environment at what’s known as “cabin altitude”—usually between 6,000 and 8,000 feet. This is much more comfortable for passengers and helps to mitigate the effects of altitude on the body, such as fatigue and dehydration, but before all, it makes possible to breathe normally.
Time of Useful Consciousness (TUC): Why It Matters
In the event of a sudden loss of cabin pressure, the time you have to act—known as the Time of Useful Consciousness (TUC)—is very short, often just a matter of seconds at high altitudes. This is why it’s crucial to put on your oxygen mask immediately. As the safety briefing advises: “Put on your own mask first before assisting others.” After all, you can’t help anyone if you’re unconscious!
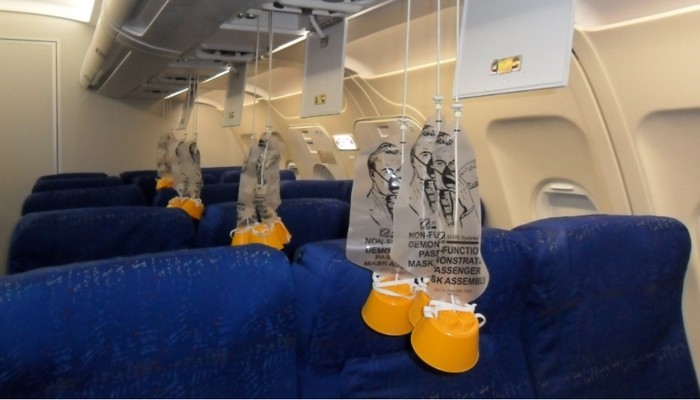
Emergency Oxygen Systems: How They Keep You Safe
If cabin pressure is lost, oxygen masks deploy automatically, providing a mix of ambient air and supplemental oxygen. For higher altitudes, the masks deliver 100% oxygen, enough to keep you conscious until the pilot can descend to a safer altitude where the outside air pressure is sufficient for normal breathing. The system is designed to supply oxygen for about 12 to 20 minutes—enough time for the aircraft to make an emergency descent.
How Pressurization Systems Work
Pressurization is managed using bleed air from the engines. This compressed air is cooled and filtered before being released into the cabin. The pressurization system carefully controls the outflow of air through outflow valves to maintain the desired cabin altitude. The injection of bleed air is constant and the regulation happens at the outflow. This system works in conjunction with the aircraft’s environmental control systems, which regulate temperature and humidity, ensuring a comfortable journey from takeoff to landing.
Safety Features and Pressurization Limits
Aircraft are designed to withstand specific pressure differences between the inside and outside environments. Safety valves prevent over-pressurization by allowing excess air to escape if the internal pressure gets too high. In the unlikely event of rapid decompression, the outflow valves close automatically to help maintain as much pressure as possible.
Historical Incidents: Why Pressurization Is No Joke
There have been notable incidents where pressurization issues have led to serious consequences. One well-known case is the 2005 Helios Airways Flight 522 accident, where a loss of cabin pressure led to the incapacitation of the crew and passengers, ultimately resulting in the crash of the aircraft. The report and the wikipedia page are not easy to read, but if you want, it is available here. Such incidents highlight the critical importance of functioning pressurization systems and emergency procedure.
Impact on the Human Body
Beyond hypoxia, high altitude and rapid decompression can lead to other physiological issues, such as barotrauma (damage caused by pressure changes), especially at the eardrums, and decompression sickness (caused by nitrogen bubbles forming in the blood). These conditions underscore the importance of maintaining cabin pressurization and adhering to safety procedures during flight.
Conclusion: Pressurization Keeps Us Safe
Next time you’re on a flight, take a moment to appreciate the invisible systems at work. Pressurization isn’t just about comfort—it’s a vital part of keeping you safe at high altitudes where human life simply isn’t sustainable. And remember: if those masks drop down, put on your own mask first—because you can’t help anyone if you’re not breathing!
Leave a Reply